Introduction
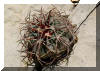
Epidermis
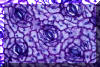
Cortex
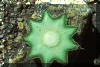
Leaves
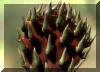
Spines
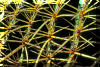
Poisons
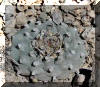
Wood
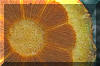
Root Succulence
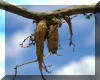
Apical Meristems
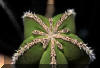
Cephalia
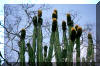
Flowers
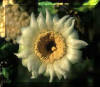
Travel
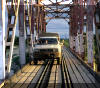
Published papers
| |
Modifications to Cactus Wood
Wood within Cactaceae is amazingly varied. It might be safe to say that no other
family has as many types of wood present within it.
Ordinary fibrous wood
Many cacti have a rather ordinary fibrous wood similar to that in many dicot
trees. The wood has a ray system produced by ray initials and an axial system
produced by fusiform initials. The rays contain just ray parenchyma cells
(although these are often sclerified, having lignified secondary walls) and the
axial system is composed of small clusters of vessels, a bit of paratracheal
parenchyma and a matrix of xylem fibers. This type of wood occurs in all
Pereskia (the leafy trees), and all cacti with such tall, slender bodies
that they must have a strong wood to support themselves. The only unusual
feature of this wood is that in virtually all examples that have been studied,
the fibers are septate and nucleate: they remain alive rather than dying once
they have completed their morphogenesis. The rays vary somewhat in height and
width and occasionally have cells with rather thick, lignified walls, but the
rays are not sufficiently abundant or voluminous to store large amounts of water
within the wood. Similarly, the axial parenchyma is limited to just a few cells
in immediate contact with vessels (technically known as “scanty paratracheal
parenchyma”). The second basic type of axial parenchyma in wood (“apotracheal
parenchyma,” surrounded by fibers and not in contact with vessels) does not
occur in cacti with fibrous wood.
Evolutionary increase in the size of rays
An overriding trend in the evolution of cactus wood has been an increase in the
amount of water stored within the wood itself. Such water is closer to vessels
than is the water stored in the cortex or pith. Increased water-storage capacity
in wood has been achieved by different means in different evolutionary lines. In
several genera, rays have become gigantic, being several centimeters tall and
several millimeters wide: large enough to be easily visible to the naked eye.
Assuming that vessels are many centimeters long (perhaps even a meter long),
each vessel could have extensive contact with several of these large rays. The
water stored within these bodies is present as a three-dimensional reserve with
the cortex, pith, rays and paratracheal water all being interconnected and
available to vessels.
Evolutionary increase in the amount
of axial parenchyma
Although some cacti have increased the size or number of their rays, in other
species it is mostly the paratracheal parenchyma that has increased in volume.
This has occurred to the point where vessels and vessel clusters are now
surrounded by abundant paratracheal parenchyma. During times of water stress,
vessels do not have to rely on the roots’ ability to extract water from the
soil, the vessels can instead quickly and easily pull water from the parenchyma
cells that encase them: cavitation should be almost impossible. And if
cavitation does occur, the abundant paratracheal parenchyma should be able to
refill the vessels. The increase in axial parenchyma is usually accompanied by a
decrease in xylem fibers, such that the wood becomes both heavier and weaker;
because of this, parenchymatous
wood usually occurs in shorter, broader plants in which some of the weight is
supported by turgor pressure. Very rarely, the wood matrix consists of just
xylem parenchyma with no fibers at all: vessels in plants with such wood
traverse nothing but water-storage tissue, all cells are filled with water and
the plant would probably die of desiccation before its vessels could possibly
cavitate (but this has not been studied).
Alterations in vessel population
characters
In
addition to having water-storage parenchyma near vessels, the wood can be
protected from cavitation by altering the ratio of wide vessels to narrow ones.
Within any particular plant subjected to water stress, wide vessels tend to
cavitate first, narrow ones last. It might seem that a desert-adapted wood
should have only very narrow, cavitation-resistant vessels, but narrow vessels
have low conducting capacity and have high friction. In contrast, wide vessels
can conduct a great deal of water with low friction but they cavitate easily.
Which should a plant use? Or should it use a mix? This has been studied in cacti
by Josh Stevenson, who developed the concept of the VC50 and VC90; these are the
percent of vessels that still are water-filled and functional when the plant has
suffered enough drought that cavitation has caused it to lose 50% or 90% of its
conducting capacity. With a high VC90, for example, narrow vessels dominate the
wood, and as drought begins to be serious, the widest vessels will cavitate
first. But because they are so rare, their cavitation does not cause a great
loss in water conducting capacity. As the drought continues, narrower and
narrower vessels cavitate, but even after 90% of all conducting capacity has
been lost, plants with a high VC90 will still have a large number of very narrow
vessels left – they are too narrow to conduct much water (but there is not much
water available to conduct), and probably that water goes to important areas
such as buds and apical meristems (but that has not been tested yet). This
appears to be adaptive for cacti. But in plants with a low VC90, wide vessels
dominate the wood, and this wood can conduct large amounts of water easily when
it is available. But drought will cause the wide vessels to cavitate, and
because they are so abundant, the plant will soon have lost use of most vessels
-- the only ones that remain water-filled and functional are the rare narrow
vessels.
The plant may still face months of drought with only a few vessels left; this
does not seem optimum for cacti, to put it mildly.
Stevenson, J. F., and J. D.
Mauseth. 2001. Cavitation and embolism repair in the dimorphic wood of
Rhipsalis lumbricoides (Cactaceae). Haseltonia 8: 51 – 57.
Stevenson, J. F., and J. D.
Mauseth. 2004. Effects of environment on vessel characters in cactus wood. The
International Journal of Plant Sciences 165: 347-357.
Mauseth, J. D., and J. F.
Stevenson. 2004. Theoretical considerations of vessel diameter and conductive
safety in populations of vessels. The International Journal of Plant Sciences
165: 359-368.
Wide-Band Tracheids (WBTs)
An
unusual feature in the evolution of cacti is the production of a cell called a
wide-band tracheid (WBT). These are short, broad tracheids whose secondary wall
has either an annular or a helical pattern but is never scalariform, reticulate
or pitted. The “wide-band” in their name comes from the fact that deposition of
the secondary wall does not stop once the wall is 1 or 2µm thick but instead
continues until the wall almost occludes the lumen. In WBTs with annular
secondary walls, it is not unusual to encounter cells with such a narrow lumen
remaining that water conduction would be almost impossible.
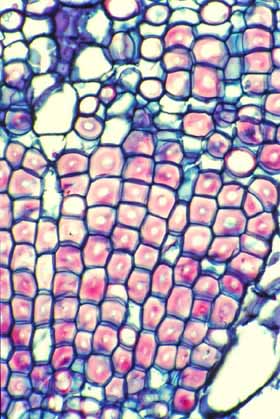 |
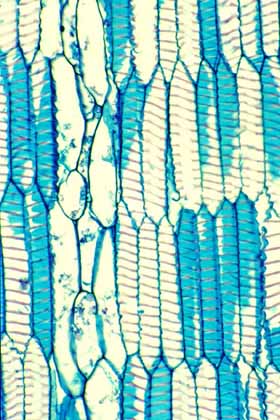 |
|
This cross section of wood of Pelecyphora
aselliformis may appear to consist of mostly fibers, but the cells
with thick walls are Wide-Band Tracheids (WBTs).
Click on any photo for a large image. |
This wood tangential section shows WBTS in
vertical view. The secondary wall is present as a helix of very wide,
band-like wall material. The tracheids here are fully expanded, but if
they were to lose water during a drought, they could shrink to a shorter
volume, the helix would become shallower, and because the cells have
smaller volumes, they would be unlikely to cavitate. |
|
The main role of WBTs appears instead to be water storage. Because the secondary
wall is either annular or helical, most of the primary wall is unlignified and
unobstructed so water can enter and leave the cell easily, but more importantly,
the rings of the annular type and the helix of the helical type do not hold the
WBT to a rigid, fixed length. As the cell loses water, the thin, flexible
primary wall can simply bend inward between the rings or gyres of the helix and
the cell becomes shorter. No matter how much water the WBT gives up to
surrounding tissues, its own volume can shrink to match the volume of water
left. Of course, a cell can cavitate only if it contains a volume of water less
than its own rigid volume. By shrinking, the cell avoids cavitation. But for
dead cells, what is the advantage to avoiding cavitation? By avoiding
cavitation, they maintain complete hydrogen-bonding throughout the mass of
water. Once rains come again and these plants have water, they also have
completely intact, hydrogen-bonded water columns that can be pulled upward by
water tension. A nice touch is that these cells then re-expand to their original
length. Obviously, WBTs can shrink only if the wood itself can shrink, so WBTs
are found almost exclusively in woods that completely lack fibers. The best bet
for finding WBT wood in cacti is to find any globose cactus whose weight is
supported by turgor pressure: as these plants lose water in a prolonged drought,
their WBTs can shrink and avoid cavitation and their entire bodies can shrink
because of their tubercles (the pleated surface mentioned on the Cortex page of
this web site). Notice the
interaction of so many modifications: pleated surface, thick water-storing,
weight-supporting cortex vascularized by cortical bundles, wood that is free of
fibers but which contains WBTs and abundant parenchyma.
The evolution of WBTs in cacti added another element to wood organization and
made possible an especially wide diversity of woods. In some species, wood now
consists of narrow tangential bands of vessels and paratracheal parenchyma
alternating with thicker bands of pure WBTs. This wood has the appearance of
having growth rings with earlywood consisting of vessels and parenchyma,
latewood consisting of WBTs. Variations on this theme still have earlywood of
vessels and parenchyma but latewood consisting of either a mixture of WBTs and
apotracheal parenchyma cells or even WBTs, vessels and parenchyma cells. A
problem with this theory is that often a plant that appears to be many years old
will have too few “annual rings.” It could certainly be that these are growth
rings of early- and latewood but the plants simply do not make a ring each year.
It is well-known that desert trees occasionally fail to make growth rings during
unusually dry years, but these cacti have so few rings it would appear that they
make wood only during exceptionally good years. This needs to be examined under
carefully controlled conditions in which the age of the plants is well-known.
WBTs occur in all three subfamilies. They are very rare in the Pereskioideae
with their hard trunks with fibrous wood, but are almost universally present in
Opuntioideae and Cactoideae. In Cactoideae, WBTs have a distribution that would
be expected of a tracheid: they occur only in the axial system of the wood (that
part produced by fusiform initials), not in the rays (they also occur in the
primary xylem of stele bundles and in cortical and medullary bundles). But their
distribution in Opuntioideae is often not at all typical of that of a tracheid:
they frequently occur in the axial system where they “should” be, but in many
opuntioid species they occur in the rays as well – rays being defined as consisting of
short cells produced by ray initials and lying opposite the rays in the
secondary phloem. In other species they occur ONLY in the rays, not at all in
the axial system, and in more than half the species, they occur in the
perimedullary zone (the outermost region of pith and innermost regions of the
medullary rays). This may seem like merely a puzzling curiosity, but I do not
know of any other group of plants that have tracheids or vessels occurring
outside the xylem.
Mauseth, J. D.,
Y. Uozumi, B. J. Plemons, and J. V. Landrum. 1995. Structural and systematic
study of an unusual tracheid type in cacti. Journal of Plant Research 108: 517 –
526.
Mauseth, J. D.
2004. Wide-band tracheids are present in almost all species of Cactaceae. The
Journal of Plant Research 117: 69-76.
Phase change in wood of cacti
A
really amazing feature of the wood of almost all members of Cactoideae is that
it undergoes a phase change. This means that for the first part of its life, a
stem or root produces one kind of wood, at a later stage of its life it produces
a different kind of wood. In almost all (but there are a few exceptions) species
of Cactoideae, once a seed germinates and begins to produce wood, it produces
WBT wood: it has an axial system of vessels, parenchyma and WBTs but no fibers
at all and its rays are very soft parenchyma cells. At some point it undergoes
its phase change and begins to make wood in which the axial system is dominated
by fibers rather than WBTs. At the same time, vessel characters may change also,
and ray cells in the second phase often have thicker walls than those in the
first phase. The change can be very abrupt, or it can be gradual with at first
the production of a few clusters of fibers near (but usually never touching)
WBTs, then more fibers and fewer WBTs until finally no more WBTs are ever
produced.
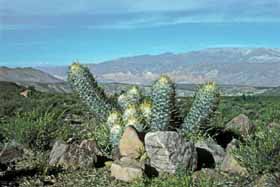 |
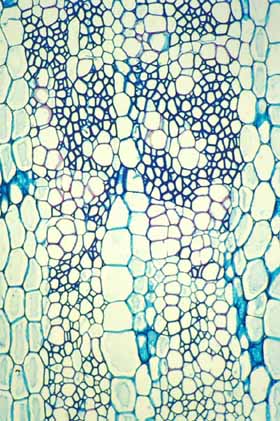 |
|
Shoots of this Oreocereus trollii will
never grow to be taller than about 40 cm; while still small, they are
short and wide enough that WBT wood will support them, but once they reach
this size, the longer shoots must switch to making fibrous wood: each
shoot makes two types of wood in sequence.
Click on any photo for a larger image. |
This wood cross section shows a weak wood at the bottom
(near the pith, produced when the shoot was young) and stronger wood at
the top (near the vascular cambium, located beyond the top of the photo).
The strong wood was produced when the shoot was older, taller and heavier. |
|
We
have no idea what the stimulus is that triggers the change, but it may be
related to weight stress. In species whose adults tend to be large and heavy or
long and slender, the phase change occurs while the plants are still young and
small, less than 10 or 20 cm tall, but which are so slender they tilt and put
stress on the wood. Conversely, in species whose seedlings and adults are
globose, the broad, squat bodies are always supported by turgor pressure, there
is no tilting and there is never any phase change at all, the plant produces
only WBT wood its entire life. For species whose adults are of intermediate
height, the initial phase of WBT wood is prolonged but not permanent: apparently
they produce WBT wood as long as they are short and wide enough to be supported
by turgor, but once they finally grow so tall that they begin to lean and must
have the skeletal support of strong wood, they switch to making fibrous wood
(this is only a hypothesis and has not been tested to verify it). A transverse
section at the base of an old plant would reveal WBT wood in the center, fibrous
wood near the periphery; a transverse section near the apex would reveal only
fibrous wood.
One of
the themes of this website is the complexity of cactus evolution, and wood
dimorphism offers another example. In the genus Samaipaticereus, wood
dimorphism runs in the opposite direction: young shoots have hard fibrous wood,
older ones have just parenchymatous wood without fibers. So, how is it that an
older plant needs less support than a younger one? Samaipaticereus is
basically a “support parasite:” it has long slender stems that must be supported
by a hard, fibrous wood, but it grows in forests where its long stems soon
extend upward into the branches of shrubs and trees. At this point, if it
happens to bend too much, it simply ends up leaning against a tree branch and
thus no longer needs to support itself, the tree is doing that. At that point,
it is advantageous for it to stop expending so much carbohydrate making
unnecessary wood fibers, and instead it just makes parenchymatous wood,
basically just vessels. This is another point that needs to be documented: we do
know for certain that Samaipaticereus has this form of dimorphic wood,
but we do not know if it is triggered by sensing that it is leaning against a
tree branch or if it just switches to making soft wood and once it falls, it
probably falls on something that can hold it up. This is perhaps not truly a
case of being a “support parasite” but rather it might be a mutualism: it could
be that the tree is protected from browsers by having a mass of spiny
Samaipaticereus branches tangled up with its own otherwise edible twigs and
leaves.
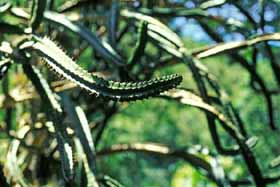 |
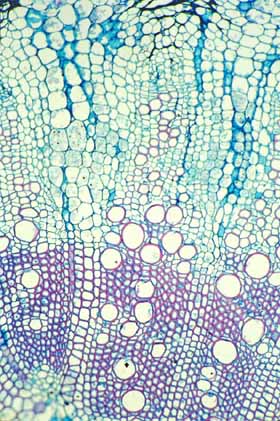 |
|
These shoots of Samaipaticereus corroanus
are long and slender and they have a wood that is too weak to hold
themselves up. Instead, they are intermingled with -- and supported by --
the branches of a tree. Once the tree begins supporting the
Samaipaticereus, it no longer has to produce strong, fibrous wood.
Click on any photo for a larger image. |
This is the type of wood found in
Samaipaticereus: it produced a strong fibrous wood while growing upward
toward the tree's branches but it then changed and produced only
parenchymatous wood after the tree began supporting it. This wood is from
Werckleocereus glaber, another species that has the same growth
habit as Samaipaticereus. |
|
Mauseth, J. D.
1993. Water-storing and cavitation-preventing adaptations in wood of cacti.
Annals of Botany 72: 81 – 89.
Mauseth, J. D.,
and T. Fujii. 1994. Resin-casing: A method for investigating apoplastic spaces.
American Journal of Botany 81: 104 – 110.
Mauseth, J. D.,
and B. J. Plemons. 1995. Developmentally variable, polymorphic woods in cacti.
American Journal of Botany 82: 1199 – 1205.
Mauseth, J. D.,
and B. J. Plemons-Rodriguez. 1997. Presence of paratracheal water storage tissue
does not alter vessel characters in cactus wood. American Journal of Botany 84:
815 – 822.
Mauseth, J. D.,
and B. J. Plemons-Rodriguez. 1998. Evolution of extreme xeromorphic characters
in wood: A study of nine evolutionary lines in Cactaceae. American Journal of
Botany 85: 209 – 218.
Arnold, D. H.,
and J. D. Mauseth. 1999. Effects of environmental factors on development of
wood. American Journal of Botany 86: 367 – 371.
Mauseth, J. D.
1999. Anatomical adaptations to xeric conditions in Maihuenia
(Cactaceae), a relictual, leaf-bearing cactus. The Journal of Plant Research
112: 307 – 315.
[End Wood page]
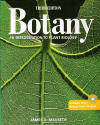
|